Acid deposition represents the mix of air pollutants that together lead to the acidification of soils and freshwaters. The term encompasses the popular idea of "acid rain", but also includes the direct uptake of pollutants by the ground in the absence of rain. This direct uptake is referred to as "dry deposition", while precipitation inputs are referred to as "wet deposition". In addition, the direct impaction of cloudwater on hills is sometimes referred to as "occult deposition".
Sulphur (S) emissions are no longer the main cause of acidification, however there may be a legacy effect. Since the deposition of nitrogen oxides and ammonium also lead to acidification, and these emissions are declining much more slowly, acidification is still a cause for concern, albeit much reduced from the days of high SO2 emissions.
What is it?
Acid deposition represents the mix of air pollutants that deposit from the atmosphere leading to acidification of soils and freshwaters. It mainly consists of pollutants emitted by the combustion of fossil fuels (e.g. power generation). The removal of these pollutants from the atmosphere is in the form of wet deposition in rainfall, cloud-water or occult deposition, mist and dew, but also includes dry deposited acidifying gases. Rainfall that is acidic, possesses elevated H+ ion concentrations, and has been commonly known as ‘acid rain’.
Today, the contribution from SO2 is considerably smaller than during the 1970s, so that currently, oxidised and reduced nitrogen dominate acid deposition. Deposition of reduced nitrogen (N) compounds, e.g.NH4+, can acidify via microbial transformations in the soil and also assimilation in plants leading to acidification of the rhizospehere. Other compounds also contribute to acid deposition, e.g. hydrochloric acid (HCl)
Acidification
Strictly speaking, not all components comprising acid deposition are acidic, although they have an acidifying effect on soils and freshwaters. For example, sulphate, nitrate and ammonium are not in themselves acidic, but can be associated with acid protons that can be released through transformations in the soil or on leaf surfaces, e.g. via oxidation, nitrification, mediated by microbes and nitrifying bacteria. Anions, negatively charged ions such SO42- and NO3-, can also acidify through the mobile anion effect: anions are poorly retained by soil, and as they move through the profile, strip off base cations, exhausting the buffering capacity so that eventually toxic acid cations such as Al3+ and H+ come to dominate exchange sites.
In soil, processes causing acidification and the consequences of acidification are linked and feedback on each other. The N status of a site can affect certain soil transformation rates and uptake patterns which drive the acidification process, e.g. nitrification - the transformation of ammonium to nitrate which generates acidity (2 protons, H+ ions). This process depends on the availability of ammonium ions for transformation by microbes. Thus the longer a soil is subject to enhanced reduced N (NH4+) deposition the greater the risk of acidification via nitrification.
NH4+ + 2O2 → NO3- + H2O + 2H+
N transformation processes represent key sources and sinks for protons, H+ ions.
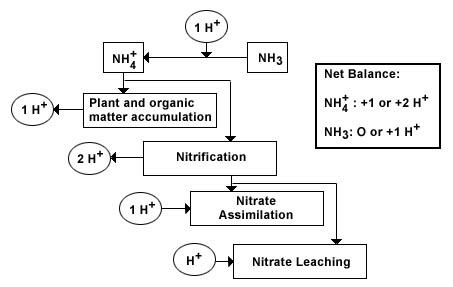
Figure 1: Effects of reduced N, NH3 or NH4+ inputs on soil proton balances. The production of H+ depends on the form of NHx input and the fate of the NH4+ ion. Diagram modified from Binkley and Richter (1997).
Acidifying deposition associated with sulphur continues to fall so that today most acidification effects are associated with nitrogen deposition.
Emissions & Deposition
Emissions of primary pollutants contributing to acid deposition have reduced substantially since the 1980s, mainly in response to international control measures (e.g. UNECE Convention on Long-range Transboundary Air Pollution (CLRTAP), and, more recently, the EU National Emission Ceilings Directive (NECD)). Detailed estimates of the spatial distribution of concentration and deposition of pollutants have been provided by Atmospheric Transport Models (ATMs). From 1970 to 2010 SO2 emissions decreased by 94% whilst ambient rural concentrations reduced by 90% between 1987 and 2008 (RoTAP, 2012).
Concentrations of acidity and sulphate in rain declined on average by 85% and 75% respectively over the last 20 years, with the decline in sulphate accounting for 75% of the decline in acid deposition. However, this decline is not equal over the whole of the UK. Deposition in areas remote from sources has fallen by disproportionately smaller amounts than deposition close to sources, e.g. central and eastern England, reflecting the smaller decline in wet deposition (-57%) compared with dry deposition (-93%). International shipping emissions have emerged as a significant source of S and N, contributing 18% and 19% to the emissions of oxidised N and S during 2005, but these emissions should fall significantly by 2020 with implementation of the MARPOL convention. Between 1986 and 2006 S deposition decreased by 80% whereas N deposition has changed little. Reduced N is expected to dominate acid deposition chemistry in the future.
Effects
Acid rain was responsible for large-scale damage to aquatic ecosystems and forests in Scandinavian countries, south eastern Canada, north eastern United States, south west Scotland (Galloway), Wales and Germany. Gaseous precursors, such as acid gases, can also cause direct damage to sensitive vegetation when they deposit in sufficiently high concentrations, e.g. SO2 damage to sensitive lichens. High altitude forests are especially vulnerable as they are often surrounded by clouds and fog in which acid ions are more concentrated. In coastal areas concentrated sea mists have caused lesions on leaves (Maine/Canada). Once the pH falls below 3.0 the likelihood of visible injury lesions, ‘burning’ and premature senescence and leaf fall increases (Cape 1993).
- Many effects of acid deposition are indirect, associated with acid deposition lowering soil pH and increasing solubility of toxic Al3+ ions, which is often associated with reduced base cation concentrations. Leaching of base cations, especially magnesium from soils, have been linked to leaf chlorosis, a common symptom on trees in some German forests in the 1980s, where this yellowing was associated with forest decline (Huettl et al 1990). Decomposition rates can be reduced in acid soils which will mean nutrient availability is compromised as mineral nutrients remain immobilised. Acid deposition can lead to calcium being leached from conifer needles, e.g. red spruce (Borer et al 2005), which become less able to withstand winter freezing / desiccation damage. The effect on food crops is minimised by the application of lime and fertilizers to replace lost nutrients and maintain a more neutral soil pH.
Reproduction in both birds and plants appears to be sensitive to acidification, e.g. egg shell condition and seed production, viability and germination. Eggshell thickness often declines as the availability of calcium in the diet falls in response to acid leaching (Nybx et al 1997; Ramsay and Houston 1999). Many crustaceans cannot inhabit acidified waters due to the low availability of calcium (Havas and Rosseland 1995; Muniz 1990).
Effects: Soils
Evidence from monitoring programmes (National Soil inventory (NSI), Countryside Survey, Envrionmental Change Network of sites (ECN), Inter-National Co-operative Programme (ICP)) and long-term experiments have shown:
- Soil acidification will be exacerbated in areas of high rainfall.
- Chemical changes leading to reduced fertility and nutrient deficiencies, e.g. lower P and base cation availability and loss of Molybdenum (Mo).
- Release of toxic metal ions, Al3+ and manganese (Mn).
- Changes in microbial transformations e.g. N transformations; low pH promotes nitrous oxide emissions during nitrification and denitrification.
Effects: Freshwaters
- The main chemical contributors to acid deposition to surface waters are non-marine sulphate and nitrate (acid anions).
- The most pronounced in upland lakes and streams remote from intensive agriculture and point source discharges. Here organisms are adapted to water of low alkalinity, ionic strength and nutrient concentrations.
- Sensitivity is highest for waters draining peats and acid soils overlying acid bedrock with low weathering rates, e.g. granites, sandstones, greywackes and schists. Geologically sensitive areas include Dartmoor, Exmoor, Snowdonia and the Cambrian mountains of central Wales, the Pennines, the North York moors, Lake District, Galloway, the Trossachs, Scottish Grampian mountains and the mountains of Mourne.
- Waters begin to acidify once base cation buffering is exhausted, through deposition of mineral acids, and replaced by increasing amounts of H+ and Al3+ ions.
- Al3+ ions can be toxic to a range of aquatic fauna.
- Reduced pH and alkalinity reduces viability of a range of aquatic plants from algae to macrophytes.
- Unlike sulphate, nitrate levels could remain high or rise even if N deposition falls. This is because ammonium ions can be converted into nitrate ions: oxidised, nitrified by microbes, both in the soil and on the surface of foliage.
- Acid neutralising capacity has been related to biota, a critical limit was defined as 20 µ eq l-1 above which long-term decline in brown trout populations would not be expected. At an Acid Neutralising Capacity (ANC) of zero, trout populations fell in 50% of Norwegian lakes (Lien et al 1996). However, high (Dissolved Organic Carbon) DOC concentrations can compromise ANC and relationships with aquatic organism health can vary over the course of the life cycle, making ANC an unreliable guide to ecological protection.
- Loss of acid-sensitive species at all trophic levels including primary producers, macrophytes and top predators, e.g. fish and water fowl, results in reduced biodiversity. Strong acidity increases the solubility of Al ions which can be toxic to fish, an issue for some Scottish lochs in the past.
- Wide ranging effects, e.g. fish, high concentrations of both H+ and Al3+ ions, can disrupt egg production, hatching success, osmo-regulation and gill function.
- There is a legacy effect associated with base cation depletion and pollutant accumulation in soils which means surface waters are still vulnerable to acid episodes.
Effects: Vegetation
- In the Netherlands acidification effects have been reported with some perennial herbs, e.g. Arnica on lowland heaths, becoming less common in response to the accumulation of NH4+ in soil as nitrification is less effective as the soils acidify (De Graaf et al 1998).
- Geology and soil chemistry influence susceptibility of an ecosystem to acid deposition; communities growing on acid soils with high aluminium content are most at risk.
- The areas most sensitive to acid deposition are characterised by minerals such as granite, gneiss and quartz rich rocks that contain very little lime (CaCO3) and do not weather easily.
- At low pH < 4.5, aluminium occurs as Al3+. This electropositive trivalent ion can be highly toxic, especially to fine roots (although mycorrhiza offer some protection);reduce P availability through precipitation and compete with Ca for uptake sites (Foy 1984; Rout et al 2001).
- Acidified rain will lower soil pH, increase Al solubility and base cation leaching and lead to many indirect effects associated with these consequences.
- pH influences the stability of the chlorophyll pigment. Enzymes that facilitate breakdown of chlorophyll b are active in a narrow acidity range compared with enzymes that breakdown chlorophyll a. Acidity lowers the chlorophyll a/b ratio because it favours the breakdown of chlorophyll a. Thus acidification can lead to reduced light capture and C assimilation.
Evidence of Recovery
Vegetation
- N deposition effects can make it difficult to evaluate effects of reduced S deposition in many ecosystems.
- Reductions in SO2 concentrations have led to sensitive lichens and bryophyte species recolonising former areas.
Soils
- Five major soil monitoring programmes have found increases in soil pH throughout the UK (RoTAP 2011). The ICP level II forest monitoring sites across the UK have detected evidence of recovery (Vanguloeva et al 2010).
- Rates of change are influenced by: soil type; historical S deposition; inherent soil pH (geology); rate of soil carbon accumulation; and past and continuing use of lime additions.
- Buffering by organic acids may delay recovery in organic soils.
- Increases in soil pH are largest in mineral soil as are declines in soil-water SO42- concentrations.
Freshwaters
- Review of changes since 1990 in waters affected by and sensitive to acid deposition (Monteith and Evans 2005).
- In non-forested catchments, acid neutralising capacity has increased in proportion to reductions in sulphate deposition.
- Changes in composition of the diatom community with a decline in acid tolerant species.
- Re-appearance of aquatic macrophytes
- Re-appearance of some acid sensitive mosses in some recovering streams.
- Small changes in the relative abundance of species; often characterised by the recent appearance and / or an increase in proportion of predatory species, including net spinning caddis in several lakes and stonefly and caddis in some streams.
- But, episodic acid events can still restrict the rate of biological recovery.
- But, labile Al3+, the most damaging consequence of acidification, remains substantially above pre-acidification levels in the more acidic waters and is still potentially a barrier to recovery.